Simulation of the Envelope Temperature of a Hot Air Balloon
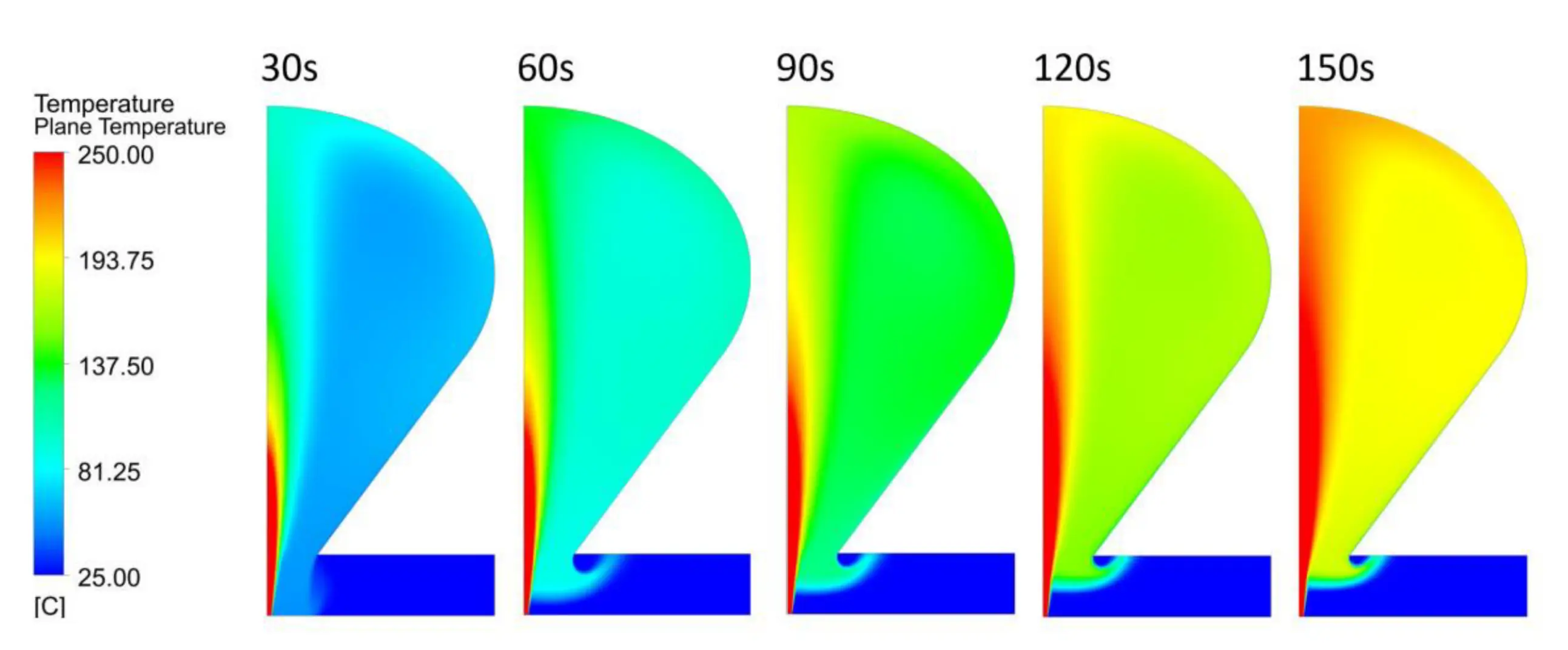
This project aims to develop a detailed physical model of a hot air balloon that describes its motion based on real weather data. In a previous project, a model for flight simulation was created. In this sub-project, the temperature, velocity, and pressure distributions are determined by computational fluid dynamics simulations and it is determined which parameters have a major influence on the aforementioned distributions.
The computational fluid dynamic (CFD) model is based on the balloon data of Stefan Zeberli's HB QZT Homebuilt. Since a hot air balloon is axisymmetric, the system can be reduced to a wedge to simplify and reduce the simulation time. The ambient pressure is used as the ambient condition. Additional model inputs for the burner are its adiabatic flame temperature and, based on the burner power, the in-flow velocity of the exhaust. Important variables for the performance of a hot air balloon are the temperature, pressure, and velocity distributions of the hot air. The internal pressure determines the buoyancy force, whereas the velocity affects the temperature distribution and thus the heat losses. The distribution of the variables can be represented time-dependently by transient simulations.
Due to large-scale natural convection flows, a steady state is not achieved. This can be seen when looking at the temperature distributions in the figure. Another goal is to find out which input variables have the greatest influence on the heat losses via the balloon envelope and opening and thus change the maximum possible flight time of the balloon. The air-fuel mixture, the burner power, the heat transfer coefficient at the balloon envelope, and the diameter of the burner are investigated. Increasing the air-fuel mixture reduces the flame temperature and increases the inflow velocity. Reducing the burner capacity results in a smaller heat quantity. The higher heat transfer coefficient increases the heat loss through the balloon envelope. Increasing the burner diameter reduces the inlet velocity, which leads to a more homogeneous distribution of the temperature inlet velocity, which leads to a more homogeneous distribution of the temperature. The CFD simulations make it possible to change the temperature, pressure, and velocity distributions of the hot air in such a way that optimized characteristics are achieved. In addition, important unknown variables such as isolation effects close to the inside of the envelope can be determined and taken into account in further calculations.